Electro-Magnetic radiation is not confined to broadcast, commercial and amateur bands, and figure 1 shows a significant portion of the electro-magnetic spectrum including the visible part. Particularly note the “fierce” logarithmic scales of frequency and wavelength. This is necessary to accommodate the huge range between “radio” waves and gamma rays in a single diagram.
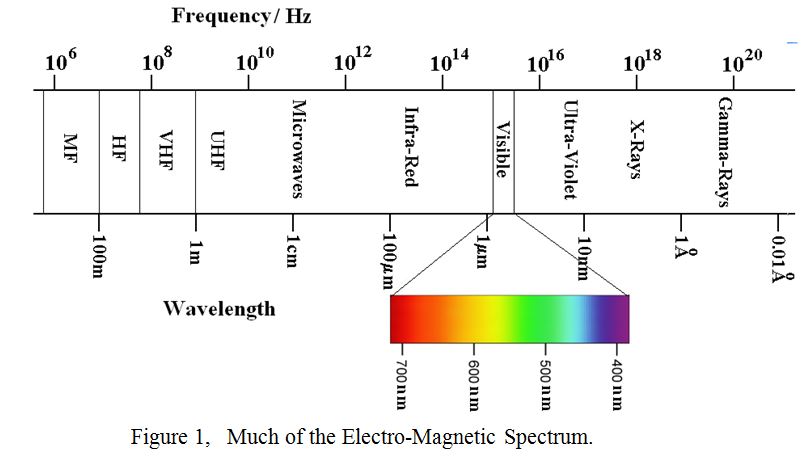
This article looks at some of the ways electro-magnetic waves are generated, starting with a résumé of the fundamentals. According to the physical laws of Gauss, Biot and Savart, and Maxwell respectively, a stationary electric charge is surrounded by, (or generates), a stationary electric field. A steadily moving electric charge generates a stationary magnetic field, and an accelerating charge generates moving electric and magnetic fields which propagate away from the direction of acceleration as an Electro-Magnetic, (E-M), wave.
The E-M waves which radio amateurs are concerned with are usually generated by carefully controlled voltages and currents applied to an aerial, and are of narrow bandwidth. However, this is not the most common case in nature and this article briefly examines the mechanisms of E-M wave production by electron acceleration, and that it sometimes leads to a broad bandwidth of radiation approximating to a continuum.
The general term “acceleration” means a change in speed, and it can be positive or negative. Negative acceleration is often called deceleration, but they both produce Electro-Magnetic waves. An example of where the accelerations and decelerations of electrons are of equal magnitude is in a simple half-wave dipole aerial. The electrons in this can be thought of as “tooing and froing” from one end to the other of the dipole. (Individual electrons do not actually move very far. It is their “influence” or “pressure wave” that moves at virtually the speed of light, but their movement from one end to the other is a useful concept for explaining their effect). The electrons, (or their pressure wave), in a resonant dipole move as a sine wave, just like the swing of a pendulum although rather faster. Now a particle moving as a sine wave is constantly accelerating and decelerating, with zero time spent not doing so, hence such movement of electrons radiates an electromagnetic wave of sine wave form. I.e. a wave of constant frequency and narrow bandwidth. Electro-magnetic waves, particularly those of the higher frequencies, are often represented as a stream of photons. This representation can be thought of as the number of electrons accelerating and hence the number of photons produced corresponding to the amplitude of the wave, and the acceleration, (rate of change of speed), corresponding to the frequency of the wave.
In the real world, such simple smooth accelerations do not always occur and we will look at some other ways in which E-M waves are produced by electron acceleration and deceleration. Acceleration of any body is often a more gradual process then deceleration. Take a motor vehicle for example. It usually takes several seconds to reach 30 mph, but the vehicle can be brought to rest in a fraction of a second by collision with a tree or brick wall. Similarly with electrons. These are often accelerated over a centimetre or two in the electron gun of a cathode ray tube, but are brought to rest, (decelerated), in the thickness of the phosphor at the screen end of the tube. In principle, even the relatively gentle acceleration in the electron gun creates an E-M wave of low frequency and very low intensity, but this acceleration is nothing compared to the deceleration in the thickness of a few atoms at the screen end of the tube. The electrons in a CRT move very fast by most normal standards. Electrons accelerated by a 4 thousand Volt electron gun travel at about 4 × 107metres per second. (Don’t try to extrapolate to their speed at higher than about 7kV because above this voltage they become increasingly “relativistic” and the extra energy goes into increasing their mass). The speed, or more accurately the energy of fast particles like electrons is measured in “electron Volts”, (eV), thus in the CRT example above, the electrons were “4keV electrons”.
When such fast electrons encounter the atoms of matter, a number of different things can happen. Now atoms can be considered to consist of a solid positively charged nucleus surrounded by ”shells” of negatively charged electrons in orbit around it. These can act as a springy cushion, such that neighbouring atoms can bounce off each other elastically. Electrons can also bounce off of atoms unless they are lucky and hit one in just the right way to knock one of its orbiting electrons into a higher orbit. The outer orbiting electrons of the atom are relatively easily displaced, but the nearer they are to the nucleus, the tighter they are bound and the harder their shells are to perturb. When electrons are pushed into a higher orbit, (of higher energy), they eventually drop back to their original orbit, (or “ground state”), with the emission of a photon. It is interesting to note that ‘heavy’ atoms are not much bigger than ‘light’ atoms as the increased positive charge in the nucleus of heavy atoms pulls the negatively charged electrons into smaller tighter orbits.
Whatever the process, a beam of electrons will be rapidly decelerated on hitting atomic matter. Some electrons will be stopped dead on their first encounter. Other may suffer a glancing blow, while yet others may penetrate several atoms and have several collisions before they stop. In general, the faster the electrons in the beam are, the further some will penetrate the atoms’ orbiting electrons. Also, the more abruptly the electrons are brought to rest, the shorter will be the wavelength of the photons produced. As an example; a 4kV electron brought to rest within the diameter of a typical atom generates a single photon of wavelength about 1 Ångstrom, Classical physics predicts that this process of “the harder the deceleration, the shorter the wavelength produced” proceeds without limit. However, Quantum theory predicts that the maximum energy of the photons produced can only equal the energy of individual electrons. Unfortunately, there is not yet any “Unified field theory” which ties classical theory and quantum theory together. However, problems in physics have no relevance to this explanation.
Because the electrons in the beam can interact with the target material in various ways from ‘dead stop at the first collision’ to multiple glancing collisions, the spectrum of photons produced is fairly wide. This wide spectrum may have superimposed upon it a sharp “line spectrum” caused by the target atom’s orbiting electrons being forced to change orbits.
In summary, the net result is that electrons of a very few eV generate visible light, or various degrees of Ultra Violet light, those of a few keV generate “soft” X-Rays, those of a few 10s of keV generate “hard” X-Rays and those of hundreds of kV to trillions of eV generate gamma rays. A medical X-Ray tube is a relatively simple device consisting of a hot cathode and an anode target all in a vacuum, (i.e. a thermionic diode), with a difference of several 10s of kV between them. The efficiency of X-Ray production, (i.e. the power of X-Ray output divided by the DC input power), is fairly low, even compared with the production of RF by sparks. However, the conversion efficiency of DC to X-Rays tends to be higher when dense heavy materials are used as the target of the electron beam because these stop the electrons quicker. This is why ‘heavy’ materials like Tungsten are used in this role.
PRAECEPTOR